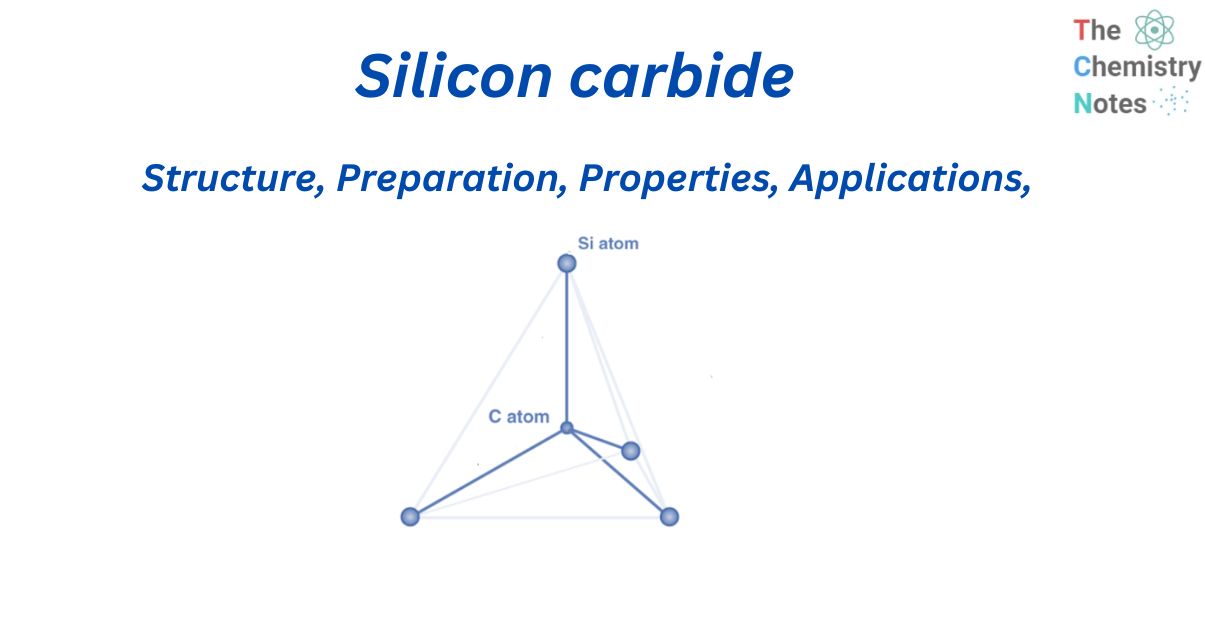
Silicon carbide is a compound of silicon and carbon. It is also known as Carborundum. Edward Acheson of Pennsylvania discovered silicon carbide in 1891. Because of its excellent combination of physical and electrical features, silicon carbide (SiC) is a wide bandgap semiconductor that is currently contributing to a profound transformation of power electronics.
SiC occurs naturally in the highly uncommon mineral moissanite. Pure silicon carbides have a colorless and transparent crystal structure. When impurities like nitrogen or aluminum are added, silicon carbide crystals turn green or blue depending on the level of contaminant. SiC is primarily utilized for its hardness and strength, but its combined ceramic and semiconductor qualities make SiC ideal for the production of high-speed, high-voltage, and high-temperature devices.
Structure of silicon carbide
Silicon carbide crystallizes in a densely packed structure that is covalently bound to each other. The Si and C atoms in a SiC crystal generate highly strong tetrahedral covalent connections (bond energy=4.6 eV) by exchanging electron pairs in sp3 hybrid orbitals.
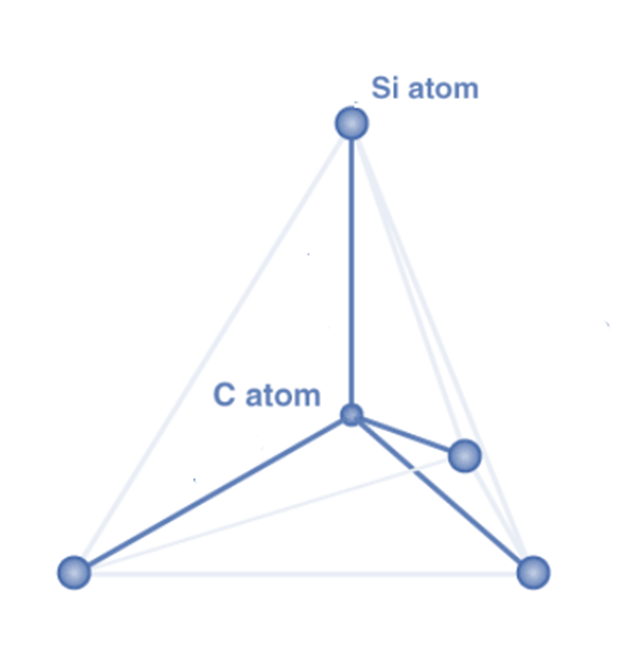
The atoms are organized so that two primary coordination tetrahedral structures are created, with four carbon and four silicon atoms bound to a central Si and C atom. These tetrahedra are connected at the corners and layered to produce polar formations. The resulting properties of SiC allow for a wide range and number of applications, particularly in adverse conditions.
Natural occurrence
While silicon carbide is uncommon on Earth, it is abundant in space. It is also a common type of stardust found surrounding carbon-rich stars, with specimens discovered in pristine condition in unaltered (basic) meteorites. Silicon carbide can also be found in space, and practically all meteorites are beta-polymorph. The Murchison meteorite, a carbonaceous chondrite meteorite, has revealed aberrant isotope ratios of silicon and carbon, indicating that these grains originated beyond the solar system.
Preparation of silicon carbide
Acheson process
Silicon carbide is present in the mineral moissanite but is uncommon in nature. It is synthesized using the Acheson process, named after its inventor, Edward G. Acheson. In this process, pure silica (SiO2) quartz sand, and finely ground petroleum coke (carbon) are combined and heated to an increased temperature of roughly 1700 to 2500°C in an electric resistive furnace. The main chemical reaction that results in the creation of ɑ-SiC is shown below.
SiO2 + 3C → SiC + 2CO
Around the core, silicon carbide forms a cylindrical ingot with layers of ɑ-SiC, β-SiC, and an unreacted material on the outside. The highest grade i.e., ɑ-SiC, has a coarse crystalline structure, and the metallurgical grade is β-SiC. SiC can be made in either green or black depending on the quality of the raw materials. The SiC ingots are next sorted and treated according to their intended application. To attain the qualities required for use, they may be crushed, milled, or chemically processed.
Lely method
Sublimation is used in the Lely method to generate bulk silicon carbide crystals. Silicon carbide powder is put into a graphite crucible that has been purged with argon gas and heated to about 2,500 °C (4,530 °F). The silicon carbide on the crucible’s outer walls sublimes and deposits on a graphite rod towards the crucible’s center, which is at a lower temperature.
Chemical vapor deposition method
Small-scale production of silicon carbide can also occur from the breakdown of gaseous or volatile molecules containing silicon and carbon in an inert atmosphere. The reaction products then deposit the carbide onto an appropriate heated substrate.
CH3SiCl ⟶ SiC + 3HCl (at 1400oC)
Properties of silicon carbide
- SiC is a light element comprised of silicon (Si) and carbon (C). Its fundamental component is a tetrahedron-shaped crystal with four carbon atoms covalently bound to a single silicon atom in the middle. SiC is polymorphic because it exists in several phases and crystalline forms.
- With a Mohs hardness value of 9, silicon carbide is the hardest substance available, followed by boron carbide (9.5) and diamond (10). Because of this apparent feature, SiC is a great material choice for mechanical seals, bearings, and cutting tools.
- Because of its fracture properties, silicon carbide crystals are highly helpful in grinding wheels and abrasive paper and cloth products.
- SiC is valuable in the construction of high-temperature bricks and other refractories because of its strong thermal conductivity, high-temperature strength, low thermal expansion, and resistance to chemical reactions.
- When SiC is refined, it behaves like an electrical insulator. However, by controlling impurities, silicon carbides can display semiconductor-like electrical characteristics. Doping with various amounts of aluminium, for example, results in a p-type semiconductor.
- SiC is a chemically inert and stable substance that has a strong corrosion resistance even when exposed to or boiled in acids (hydrochloric, sulfuric, or hydrofluoric acid) or bases (concentrated sodium hydroxides). It is discovered to react in chlorine, but only at temperatures of 900°C or above. When the temperature is around 850°C, silicon carbide will begin an oxidation reaction in the air to generate SiO2.
Advantages of silicon carbide
Historically, silicon carbide has been used in high-temperature applications like bearings, heating equipment components, automotive brakes, and even knife-sharpening tools. The key advantages of SiC in electronics and semiconductor applications are:
- Thermal conductivity ranges from 120 to 270 W/mK.
- Low coefficient of thermal expansion of 4.0×10-6/°C
- Maximum current density is high.
SiC has exceptional electrical conductivity due to the combination of these three properties, especially when contrasted to silicon, SiC’s more popular relative. SiC’s material properties make it ideal for high-power applications requiring high current, high temperatures, and high thermal conductivity.
Applications of silicon carbide
Cutting and abrasive tools
Because of its low cost and durability,SiC is a popular abrasive in the arts and modern lapidary. It is also utilized in the manufacturing process for its hardness in abrasive machining techniques such as honing, grinding, sandblasting, and water-jet cutting. To make grip tape for skateboards and sandpapers, silicon carbide particles are glued to paper.
Bulletproof armor
Bulletproof armor is made from SiC. The hardness of this compound allows it to be used for this purpose.
In solar industry
SiC-enabled inverter optimization also contributes significantly to efficiency and cost reductions. The use of SiC in solar inverters improves the switching frequency of the system by two to three times that of ordinary silicon. This increase in switching frequency allows for a reduction in the magnetics of the circuit, resulting in significant space and cost savings. The reliability of silicon carbide enables solar systems to reach the consistent lifetime required to operate continuously for more than a decade.
In jewelry
SiC, which is structurally similar to diamond but more lustrous, cheaper, more durable, and lighter than diamond, is a well-deserved alternative to diamond in the jewelry industry.
In automobiles
SiC can meet high voltage needs with ease. Silicon carbide has the potential to expand electric vehicle driving range by improving overall system efficiency, particularly inside the inverter system, which improves overall energy conservation while reducing the size and weight of battery management systems.
References
- https://www.britannica.com/science/silicon-carbide
- https://www.sciencedirect.com/science/article/abs/pii/B9780081020968000057
- https://www.vedantu.com/chemistry/silicon-carbide
- https://byjus.com/chemistry/silicon-carbide/
- http://accuratus.com/silicar.html