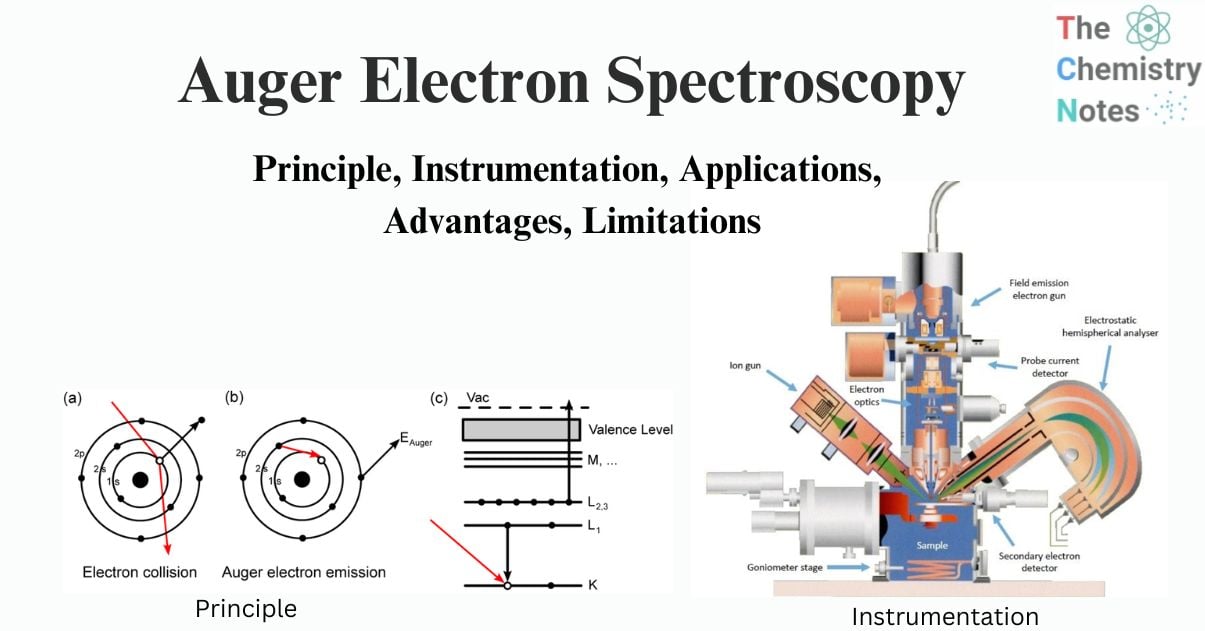
Auger electron spectroscopy (AES) is a type of electron spectroscopy that detects electrons produced by Auger relaxation processes. It is a non-destructive core-level electron spectroscopy used to determine the elemental composition of surfaces, thin films, and interfaces in a semi-quantitative manner. Auger Electron Spectroscopy (AES) was developed in the late 1960s, taking its name from the effect initially noticed by Pierre Auger, a French physicist, in the mid-1920s.
Auger electron spectroscopy has atomic number-dependent sensitivity as well, which can vary by up to an order of magnitude. The chemical shift and line shape detected in AES can provide bonding or chemical state information. However, Auger electron spectroscopy is less accurate than XPS when it comes to getting bond information. Auger electron spectroscopy has a depth resolution of 5-25, making it ideal for depth profiling with simultaneous ion sputtering. AES is a prominent surface analysis approach due to its high surface sensitivity (analysis depth of < 100 Å) and very low detection limit (0.1 atomic percent). AES can also be used to distinguish between elements that are near the periodic table.
Interesting Science Videos
The Auger Effect
The Auger effect occurs when an electron is expelled from an atom as a consequence of core-hole recombination on the same atom. When core or inner valence electrons on an atom get excited, they leave a hole in the atom’s electronic structure. Since such electrical arrangements are typically unstable, a more energetically bound electron will relax to fill the core hole. The energy needed to emit a second electron comes from the relaxation process.
Auger spectroscopy can be done in both resonant and non-resonant modes. The resulting Auger spectra in both circumstances can be highly complicated, as many distinct electrons can undergo core hole recombination and emit an Auger electron.
Principle of Auger Electron Spectroscopy
AES is based on the study of electrons ejected from an excited atom after a series of internal relaxation processes. Auger is a three-electron process in which an electron beam with an energy of 1 to 10 keV hits a solid surface. The impact of the beam ejects one electron from the surface atom’s inner or core-shell, resulting in an ionized excited atom. A non-radiative transition allows an electron from an outer shell to occupy the vacancy created in the core shell.
When a high-energy electron (or photon) strikes an atom, an inner electron is released. An outer-shell electron then fills the vacancy left behind. The energy released then causes an Auger electron to be emitted, which may be detected and analyzed. The difference between the initial transition (L1 K ) and the Auger electron’s ionization energy (L2,3) is the Auger electron’s kinetic energy. The spectroscopic designation of the energy levels involved in Auger electron emission (KL1L2,3) defines the Auger electron.
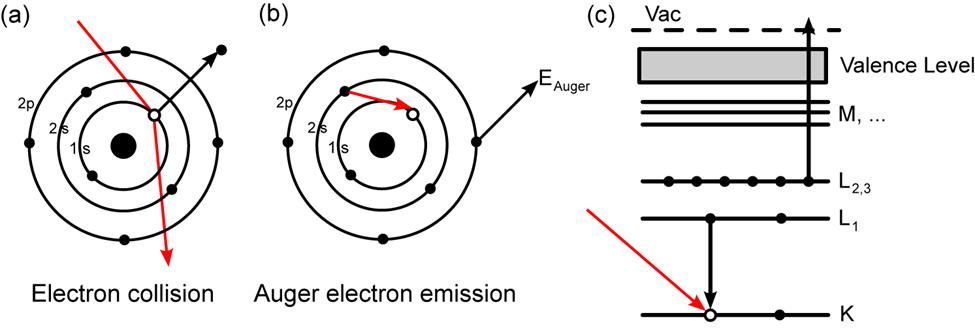
Fig: Principle of Auger Electron Spectroscopy (AES)
https://www.maths.tcd.ie/~bmurphy/thesis/thesisse1.html
As Auger uses three electrons, AES cannot be used to detect hydrogen and helium. Furthermore, despite the fact that lithium has three electrons, a solitary lithium atom in its ground state does not form Auger peaks since the atom only has two energy levels with electrons. Nonetheless, Auger peaks have been observed in multiple excited lithium atoms. Furthermore, electrons in the valence band of solid lithium enhance Auger transformations of type KVV.
Auger transition
The transitions are denoted by the letters K, L, M, and so on. This spectroscopy is primarily involved transitions like KLL, LMM, MNN, and so on. Ejection of a K-shell electron, for example, frequently leads to the drop of one electron from the L-shell electron to the core level and the ejection of the second Auger electron from the L-shell. As a result, this is known as the KLL transition.
The electronic energy levels involved in the transition are referred to as Auger electrons. An LMN Auger electron, for example, will entail initial ionization from the element’s L shell, relaxation of an electron in the M shell, and subsequent emission of the Auger electron from the element’s N shell.
Instrumentation of Auger electron spectroscopy
The main components of an AES spectrometer are
• Ultra high vacuum environment
• Electron gun
• Electron energy analyzer
• Electron detector
• Data recording, processing, and output system
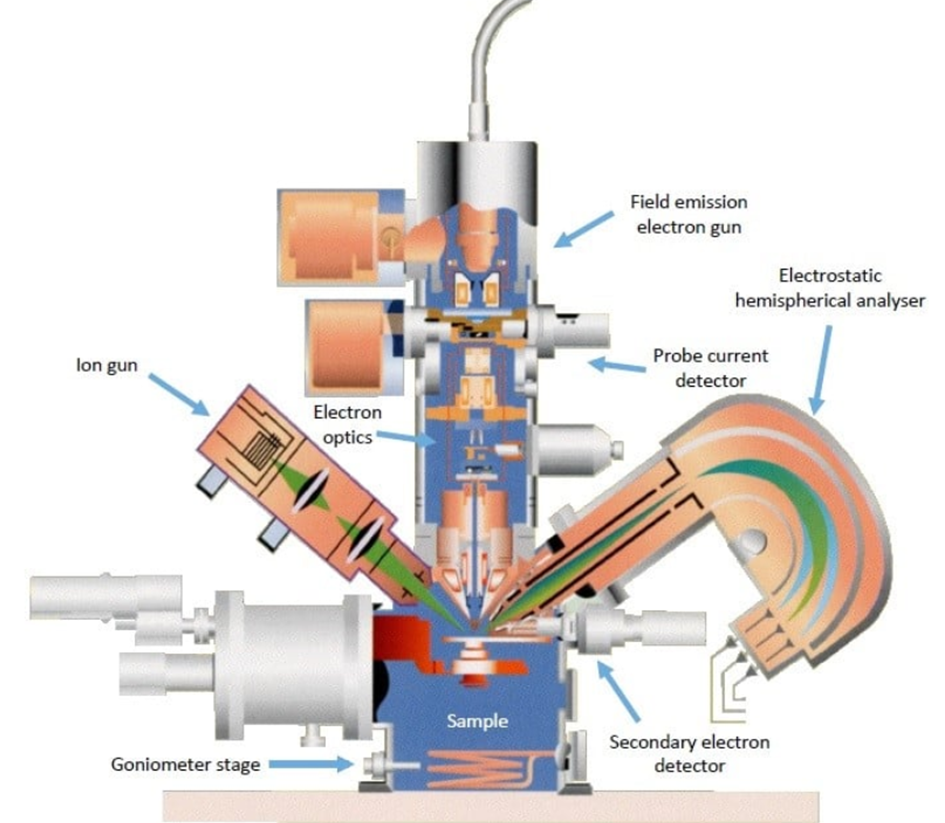
Fig: Instrumentation of Auger electron spectroscopy (AES)
image source: https://www.ntu.edu.sg/facts/equipment/detail/jeol-jamp-7830f
Ultra-high vacuum environment
Auger electron spectroscopy instruments are typically operated at pressures of 10-9 torr.
UHV is essential for surface analysis for the following reasons.
- To eliminate the sample’s absorbed gases.
- To prevent contaminant absorption on the sample.
- To avoid arcing and high voltage failure.
- To extend the mean free path of electrons, ions, and photons.
Electric gun
Auger electron spectroscopy stimulates the material surface with a focussed electron beam, yielding typical SEM pictures. These photos give spatial maps of structures or textures that can be used to define zones of interest for AES analysis. Modern AES devices often employ a Field Emission electron as the source, with a spatial resolution of 5 nanometers.
The type of electron gun used for AES analysis is primarily determined by three factors:
- Analysis speed (high beam current required).
- The preferred spatial resolution.
- Beam-induced surface modifications.
• The lower current provides excellent spatial resolution, whereas the higher current provides speed and high sensitivity in situations where spatial resolution is unimportant.
• The application of high current in particular samples may cause surface damage to the specimen and should be avoided.
Lenses
The electron lenses used to concentrate the beam might be magnetic or electrostatic. The magnetic lenses have the lowest aberrations and thus offer the highest performance.
Ion gun
The ion gun directs a focused beam of ionized gas (often Ar) at the sample to remove surface (also known as adventitious) impurities. Because the AES is generated by only a few atomic monolayers at the material surface, any sorbed gases will be detected on a material “as received” that has been in contact with the atmosphere. A rastered region is characterized as “dusting off” any surface contaminants.
Energy analyzer
Electron energy analyzers are used to calculate the number of electrons released (N) as a function of their energy (E). The most commonly used analyzers are (i) RFA or retarding field analyzers. (ii) CMA, also known as the cylindrical mirror analyzer. CHA stands for the concentric hemispherical analyzer.
RFA: The average transmission efficiency of retarding field analyzers results in inadequate energy resolution and low signal-to-noise ratios. As a result, RFA is commonly used in low-energy electron diffraction studies but is not recommended for AES.
CMA: A CMA is made up of three parts: an electron gun, two cylinders, and an electron detector. An electron gun is directed at the sample during CMA operation. The electron is then discharged into the gap between the inner and outer cylinders. The inner cylinder is at ground potential, whereas the potential of the outer cylinder is proportional to the electron’s kinetic energy. The outer cylinder’s negative potential causes it to redirect the electron in the direction of the electron detector. Only electrons falling within the solid angle cone are detected. The number of electrons found as a function of kinetic energy produces a signal that is proportional to that number. CMA is appropriate for AES because of its excellent transmission efficiency, compact size, and ease of usage.
CHA: Because of their great resolution, CHA is employed for Auger electron spectroscopy anytime chemical state analysis is undertaken. CHAs, which include an input lens and a hemispherical analyzer, are used in XPS energy analyzers. Electrons expelled from the surface pass through the input lens, which focuses and retards their energy for improved resolution. Electrons are then admitted into the hemispheres via an entrance slit. Only electrons with a narrow range of energy differences can depart the hemispheres due to an applied potential difference. Finally, the electrons are analyzed using an electron detector.
Detectors
After leaving the analyzer, the electrons reach the detector, where they are amplified and counted using an electron multiplier. These electrons are detected using a variety of detectors. Commonly used detector involves:
- Single channel detector: A channel electron multiplier detector is used in conventional instrumentation.
- It is a continuous dynode surface, which is a thin-film conductive layer on the inside of a tubular channel, that is used in an electrostatic device.
- The conditions for electron multiplication can be created with just two electrical connections.
- This detector produces a series of pulses, which pass through a pulse amplifier/ discriminator and finally into a computer.
- Multi-channel detectors: At the analyzer’s output, a multiple detection system can be implemented. The system could consist of a few multiple, parallel, equivalent detector chains or position-sensitive detectors distributed across the entire analyzer output slit plane.
Data recording, processing, and output system
All modern Auger electron spectroscopy equipment includes sophisticated computer control, data processing, and display systems. The primary functions of a computer-controlled system are gathering information and data processing.
Applications of Auger Electron Spectroscopy
- Information collected from the Auger chart provides the information of elements.
- Nanomaterials, photovoltaics, catalysis, corrosion, adhesion, semiconductor devices and packaging, magnetic media, display technology, and thin film coatings used in numerous applications are just a few examples of the industrial and research applications where the information provided by Auger electron spectroscopy about surface layers or thin film structures is crucial.
- Shifting or changing of the Auger peak gives information about the chemical shift.
- It provides information on the electronic structure of a material, such as the valence band density of states.
- Auger electron spectroscopy provides an effective chemical characterization approach for nanowires, nanocones, and other materials with technological advances in spatial resolution, high-stability imaging systems, and atomic layer depth profiling using low-energy Ar ions.
Advantages of Auger Electron Spectroscopy (AES)
- It is surface-sensitive spectroscopy and has detection limits of 0.1 at%.
- There are few matrix effects when the top few atomic monolayers (0.4–10 nm) are studied.
- When performed in survey mode, inventories of all surface components for every element of Z≥3 (Li and heavier) can be obtained.
- It aids in elemental and chemical composition analysis by comparing samples of known composition to standard samples.
- It is capable of detecting elements heavier than Li. Excellent sensitivity for light elements.
- Sub-micron maps of element distribution on material surfaces are possible to obtain. This is highly useful for determining if the composition of material surfaces is homogeneous or heterogeneous.
- It has good absolute detectability down to 100 ppm and is hence utilized for trace analysis.
- It can provide a three-dimensional map of the composition and chemistry of a sample volume.
Limitations of Auger Electron Spectroscopy
- In nonconducting samples, there is a charging effect.
- Non-conducting samples necessitate special handling.
- Analyses can only be performed on solid specimens.
- Samples that degrade when exposed to an electron beam cannot be investigated.
- Quantification is difficult.
- Hydrogen and helium are undetectable because they have less than three electrons.
References
- https://chem.libretexts.org/Bookshelves/Analytical_Chemistry/Physical_Methods_in_Chemistry_and_Nano_Science_(Barron)/01%3A_Elemental_Analysis/1.14%3A_Auger_Electron_Spectroscopy.
- Characterization: Surfaces, Interfaces, Thin Films, Butxetworch-Heinemann, Reed Publishing (USA) Inc., 1992.
- https://gyansanchay.csjmu.ac.in/wp-content/uploads/2022/01/310-L13.pdf.
- https://www1.udel.edu/pchem/C444/info/AES_XPS.pdf.
- https://www.azonano.com/article.aspx?ArticleID=6212.
- https://epgp.inflibnet.ac.in/epgpdata/uploads/epgp_content/S000831ME/P001676/M030248/ET/1525950684Module-6_Unit5_COM-I.pdf.
- https://inis.iaea.org/collection/NCLCollectionStore/_Public/28/053/28053345.pdf.